July 7, 2021 – By Tim Burch
Earlier this year, we looked back at 2020 and reviewed how surveying has dealt with the worldwide pandemic while adapting to the new tools and technology being created. We discovered the need for surveyors has not diminish during this crisis, and in many places the demand has increased significantly. Instruments, computers and measuring methods continue to increase in capability and complexity to help with the shortage of qualified field crews, yet we still need to expand our efforts to find the next generation of surveyors.
How do we find the future geospatial experts, data collectors and surveying professionals? The answer is right under our noses, and our current group of practitioners should continue to encourage aspiring students to study the geospatial sciences..
What is the word, you ask?
Technology!
Younger generations understand technology better than most practicing surveyors. New devices, methods and operations are being invented at a fast pace, and our best and brightest should be considering using that technology in a rewarding career. Before we make the big pitch to them, however, we should refresh our understanding of recent surveying history to better understand why the technology is important.
How did we get here? A short historical look at measuring
Measurement methods, devices and instruments used by surveyors have radically changed in the past 50 years, and we have covered their evolution in past columns (Survey Scene May 2016, May 2017 and Sept. 2019).
Instruments and devices used by surveyors vary in function and output of information. Some are used to physically measure the distance from one stationary point to another, determine horizontal and vertical angles at a specific location, or determine grade differentials between various points.
Other instruments are used to determine relative horizontal or vertical positions to establish locations and elevations. All these instruments are being used to gather positional data on any number of items, but the quality of the information may vary depending on the technology and method used. How?
Devices and methods for measuring distances

Tools for measuring distances have been around for centuries. The Egyptians are famous for their “rope stretchers,” while early surveyors in Europe and the New Colonies were known to use the Gunter’s chain and a measuring wheel. In the early 1800s, steel tapes were invented to replace the chain. These measuring tapes continued to evolve well into the 20th century with varying metals, fiberglass and nylon-coated plastics.
In the mid-20th century, scientists and physicists began to experiment using light waves as a means of measuring terrestrial distances. These experiments led to the development of the first electronic distance meter (EDM), commercially produced by the Swedish company Svenska Aktiebolaget Gasaccumulator (AGA) in the early 1950s. Other methods of electronic measurement, including microwave and infrared wave technology, were also developed in the years following the introduction of the light wave EDM.
For many years, the EDM was used independently from transits or theodolites to measure long distances. For those who needed to consistently measure long distances, the invention of the EDM was not just a time saver, but also provided much higher accuracy than manual measurements.
Other technologies were developed in the latter part of the 20th century, introducing the surveyor to laser scanning, but we can defer this topic until later in this column.
Devices for measuring angles

The T3 theodolite was introduced in 1925. With its 10.5-inch telescope, this theodolite had a range of up to 60 miles. It saw heavy use between 1952 and 1984. (Photo: NOAA)
The surveyor, like the astronomer, has consistently been at the forefront of developing optical instruments. The key has been combining high optical quality with a means of measuring horizontal and vertical angles within the instrument. The creation of the theodolite and the transit revolutionized the ability of the surveyor to accurately measure angles and apply trigonometric functions to determine mathematical computations. In addition, the surveyor’s compass was also developed to assist with angle measurement — with less accuracy but greater flexibility.
By the 1920s, optical theodolite technology was rapidly improving through the work of Switzerland’s Heinrich Wild. Beginning with the T2 and T3, these instruments provided accuracy and precision not previously available to the surveyor. Other manufacturers followed suit with similar instruments for the next several decades and were used in conjunction with the EDM for larger surveys. Anticipation grew with the competition to see which instrument company could marry the theodolite and the EDM into one easy-to-use, yet accurate, optical instrument.
Introducing the total station
By the late 1960s, technology had firmly entered the surveying world with a few electronic advancements. In 1968, Zeiss — a German company known for its lenses and optical systems — produced the first known tachymeter, combining a theodolite with an electronic distance meter. The tachymeter became better known as the total station, as it was capable of measuring angles and distances in one instrument. While somewhat crude and hard to use, the Elta 14 total station introduced the world to a future generation of surveying instruments that would revolutionize the field.
In the course of a few years, several manufacturers developed their own total stations. The biggest hurdle was combining the optics of the scope with the measuring axis of the EDM. By the end of the 1970s, most total stations were coaxial, therefore measuring angles and distances was done with one sighting.
Robotics were introduced in the early 1990s, with two servo motors to drive the horizontal and vertical movements of the total station. These movements were controlled remotely by the tracking system connected to the prism pole and data collector. Not requiring a human being to remain stationary and manually operate the total station provided cost savings and additional efficiency for the field crew.
Positions, everyone! Positions!

U.S. National PNT Architecture. (Graphic: U.S. Department of Transportation)
Positional measurement has revolutionized not just the surveying profession, but a large portion of everyday tasks as well. From monitoring travel times for your commute to providing your food-delivery driver with your location, position determination is the key element to these services. Satellite navigation is now the primary technology used for positioning, navigation and timing (PNT) and a big part of most aspects of surveying.
GNSS (Global Network of Satellite Systems) Positioning
Though GNSS began as a military system in the US and erstwhile USSR mainly for warship navigation, it soon became clear that there could be many applications in the civilian area.
Finding your position on a globe is a problem that has bedeviled travelers and explorers from the time it was firmly established that the earth is not flat, at least geographically speaking. The main problem is finding a point of reference and a coordinate system that can be used to orient oneself on a sphere like object like the earth. The Pole Star provided a celestial point of reference and became North; hence the opposite end became the South; and exactly in-between we placed an artifact called the equator.
The solar elevation at local noon, measured with a sextant, was converted to latitudes, but longitudes presented a big problem till an English carpenter-turned-clockmaker, John Harrison, developed a seagoing, accurate chronometer to determine the difference between local time and Greenwich time to calculate the longitude. Greenwich became longitude zero, another artefact which has since shifted westward by 100m.
From spheroid to spheroid
Sextants and chronometers are not everybody’s toys. Therefore, their use remained a matter for sea and air navigation. Air navigation also needed new tools like ADF, INS and VOR. Land navigation was simpler, but needed maps, while navigation charts were needed for sea and air navigation. It soon became clear that maps and charts, based on local models of the earth called spheroids posed a problem while moving from one spheroid to another as one navigated the globe. It required mathematical transformations to maintain the accuracy of location from spheroid to spheroid. With the advent of satellites an opportunity arose to model the earth in its entirety using very precise measurements by geodetic and ranging satellites to create a global spheroid to which all measurements could be referenced. Thus, all forms of navigation could now use one unified model, the WGS 84 being the latest in the series.

Using satellites for navigation began with the US Navy Navigation Satellite System, NNSS in 1964 using the TRANSIT satellite system. This system proved to be useful not only for the Navy, but for civilians as well. Notably, in 1983, the height of Mount Everest was corrected by the TRANSIT system to 8,850m from the original 8,840m measured in 1856. (Today it stands at 8,848m as measured by the Chinese). From 1970, the Russians had similar systems named Parus for military use and Tsikada for civilian use. Today, the US TRANSIT system has been replaced by GPS and the Russian systems by GLONASS. There are several global and regional systems for position location and navigation. Other global systems under development are Europe’s Galileo and China’s COMPASS, now called BeiDou-2 or BDS. Among regional systems are India’s IRNSS, now called NAVIC, and Japan’s QZSS.
Working of GNSS receivers
Navigation satellites work on the principle of trilateration. Position of an object is determined by its latitude, longitude on the spheroid and height above Mean Sea Level. If at the time of measurement, the instantaneous position of three satellites are known and the distance of the point of measurement from each of these three satellites is known, then the latitude, longitude and height of the point can be determined using simple distance formula. In practice, a fourth satellite is needed to adjust for timing biases.

How does a GPS receiver determine its distance from each satellite? It does so by comparing a code generated by a satellite with the same code generated internally in the receiver. The time difference between the two codes multiplied by the speed of light gives the distance. That requires a very stable signal source on the satellites which is provided by the Rubidium clock which is used to generate the code and the carrier signal for the code. Each satellite has a unique code hence the receiver can identify each satellite in its view. The carrier also contains the precise orbital parameters of the satellite which is updated regularly. The orbital parameters are updated using Integrity and Range Monitoring Stations. A central control station uploads the orbital parameters and synchronizes the Rubidium clocks regularly on each GNSS satellite.

Signals from the GNSS satellites are transmitted in the L-band in five designated frequencies named L1 to L5. Of these L1 and L2 are used by all GNSS satellites, L3 is set apart for transmission from satellites to ground stations to detect nuclear explosions. L4 is to be used for ionospheric corrections and L5 will eventually support safety-of-life applications for aviation and provide improved availability and accuracy.
Global Systems
Global Positioning System, GPS – USA
This is the oldest system which began operations in 1978 and grew to 32 planned satellites in Medium Earth Orbit, MEO of 20180 km. The orbits are so selected that at any given time at least six satellites are in view an observer anywhere on earth. Initially exclusive for military use, it was opened to the public in the mid 80s with a selective availability that restricted the positional accuracy to 100m. The military continues to enjoy 5m or better accuracy. SA was discontinued from 2000 during which time the military also developed technologies to deny GPS service on regional basis to potential adversaries. It is widely believed that this was used during the Kargil War.
GLONASS – Russia
Starting with a decision in 1978 GLONASS became operational by 1993 over Russia with 12 satellites in 2 orbits at 19,130 km. Its full capacity and global coverage was achieved by 2015 with 23 of 27 satellites operational. The accuracies are comparable to but a little lower than GPS. However, the accuracies are better than GPS in the higher latitudes. The frequencies are the same L1 and L2 but originally adopted a different access mode, FDMA before shifting to CDMA. GLONASS also works on a different spheroid model but its difference from the WGS84 is less than 40cm. Using both GLONASS and GPS together, GNSS receivers have access to 50 satellites and therefore can provide better position location faster, particularly in urban canyons and rugged mountainous areas.
Galileo – European Union
This project is being established by ESA under the leadership of Germany, France and Italy. Other European countries who have joined the project are Norway and Switzerland. Israel, Morocco and Ukraine have also joined the program. China was to have joined but later withdrew as it decided to proceed with its own BeiDou program. The program seeks to develop independent capability in the civilian space as opposed to GPS, BeiDou and GLONASS which are under military control. Galileo will have accuracy higher than GPS and GLONASS at one meter for public use and one centimeter for paid users. There is a provision for exclusive military use in case of extreme situations like war. The program was beset by funding problems as well as friction with US on security issues. However, these have been overcome and 12 of the planned 30 satellites are in orbit. Full operational status is expected by 2020.
Galileo is unique as it is designed to provide a new global search and rescue (SAR) function as part of the MEOSAR system. Apart from relaying the distress signals from emergency beacons to the rescue coordination centre, it will also provide a return link to the beacon to confirm receipt of the distress signal and launch of rescue efforts. This does not exist in the current Cospas Sarsat system.
BDS / BeiDou-2 / COMPASS – China
The Chinese system began as a regional system, BeiDou-1 with three geostationary satellites serving China and its neighborhood. The signals were in the S-band and offered an accuracy of 20m. BeiDou-1 was decommissioned by end of 2012.
BeiDou-2 is a completely new system consisting of 35 satellites of which five are planned to be in geostationary orbit to provide BeiDou-1 compatibility to existing ground systems, 25 are planned in MEO and 5 are planned in inclined geosynchronous orbits. From records of launches there are 6 in geostationary orbit, 8 in inclined geosynchronous orbit and 5 in MEO. The extra satellites in GEO and IGSO are possibly in orbit spares. The BeiDou-2 frequencies overlap the Galileo frequencies, thus providing interoperability between the two systems. As of now, the system is referred as the BeiDou Navigation System, BDS, and currently serves China and its neighborhood. BeiDou is expected to become operational globally by 2020 providing 10m accuracy for public and higher for military use.
Regional Systems
NAVIC – India
The IRNSS system, christened NAVIC after operationalization, is a direct outcome of the restrictions placed on use of the GPS system by the US during the Kargil War with Pakistan. The NAVIC system consists of 7 satellites, 3 in geostationary orbit and 4 in inclined geosynchronous orbits. The system became operational in 2016. The system works in the L5 and S bands and provides better than 20m over the Indian Ocean region and 10m accuracy over India for public use and 10cm for authorized users, which includes the military.
QZSS – Japan
The QZSS or the Quasi-Zenith Satellite System has a unique configuration of the satellite orbit. It is a highly inclined slightly elliptical geosynchronous orbit which gives coverage over Japan and the Pacific region. As of now one satellite, MICHIBIKI has been launched, three more QZSS and one geostationary satellites are in the pipeline. Using the standard GNSS L1, L2 and L5 frequencies, QZSS will complement GPS and will provide positioning, augmented positioning and messaging services. Service is expected to begin from 2018. Ultimately, four more QZSS satellites are planned by 2020. Accuracy is expected to be better than 7m. The precision service will be around 2m and will use a DGPS signal on the L band.
Satellite Based Augmented Systems – SBAS
Based on these GNSS, there are several regional augmented navigation systems under the generic name Satellite Based Augmented System, SBAS. These are Wide Area Augmented System, WAAS in the US, European Geostationary Navigation Overlay Service, EGNOS in Europe and GPS-Aided GEO Augmented Navigation System, GAGAN in India, all based on the GPS system. MTSAT Satellite Based Augmentation Navigation System, MSAS, in Japan and System for Differential Corrections and Monitoring, SDCM, in Russia based on GLONASS and GPS are others. China is planning SNAS, Satellite Navigation Augmentation System, to provide WAAS-like service for the China region. These systems are primarily meant for aircraft navigation including landing and takeoff stages but are also used for other land and sea based navigation applications. They require additional equipment to augment the basic GNSS receivers.
The SBAS is an improvement of the positioning service of the GNSS. The problem with the GNSS is the error due to the atmosphere through which the signal has to pass before reaching the user equipment like a smartphone. This error, called the tropospheric and ionospheric error introduces random variations in the signal which degrades the accuracy in the measurement of the distance of the device from the satellite emitting the signal. Using a GNSS in a differential mode like DGPS can reduce this error. But, this is not possible in a fast moving object like an aircraft. In a SBAS, there are several ground stations which continuously measure the error and update corrections through another channel, like a geostationary satellite. These corrections are applied in real time and can bring down the error to one meter allowing for a CAT-I Instrument Landing System capability.
Remote sensing
Here is where we can discuss laser scanning and other remote sensing technologies. Remote sensing is the science and technology of gathering data from a distance. Traditionally this has been mostly done from aircraft, satellites and vessels. However, technology has expanded so that most practitioners now consider the use of laser scanning, lidar, photogrammetry, hyperspectral cameras, bathymetric sonar and simultaneous localization and mapping (SLAM) to be included in the category. Keep in mind that all these technologies are types of measurements; they are not the vehicle or instruments used for the measurement.
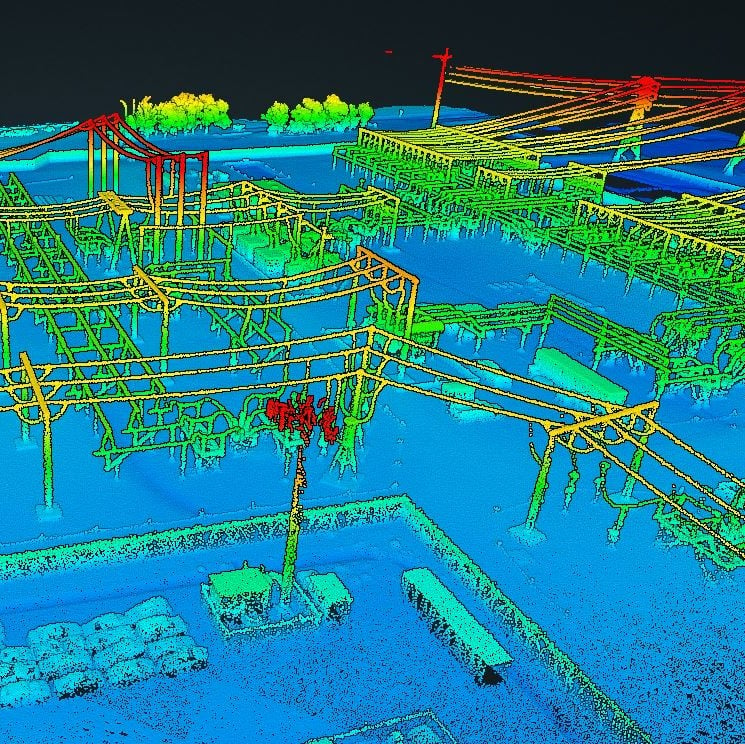
These various sensor types can collect millions of data points in a short amount of time. While surveyors are adapting to working with point clouds and gigabytes/terabytes of data, it is a radical departure from our recent past using only total stations and GNSS receivers. Significant advancements in computer processing, data storage and programming have simplified the manipulation of point clouds, but they remain a challenging task for even newer surveyors to tackle.
Autonomous vehicles
Hobbyists have been building (and crashing) model airplanes and helicopters for many years. Most of the public does not realize that the big advancement in remote-control aircraft was the introduction of GNSS technology into the flight system. Sure, we all have GNSS receivers in our phones, but now to be included in our toys? This somewhat simple addition has turned unmanned aerial vehicles (UAV) into a revolutionary tool for several occupations, not just surveyors. More control and stability of the UAV means expanded uses for emergency personnel, utility providers, parcel delivery and much more. Being able to program a specific flight provides the UAV user with higher accuracy and precision, but it takes away the element of human control.

Another vehicle gaining market share is the unmanned surface vessel (USV), used for performing hydrographic surveys. Like its UAV cousin, the USV is autonomous and is programmed to follow a specific route for greater accuracy and precision. Because of the shallow draft of a USV, it can be used in many areas deemed inaccessible by manned vessels.
An additional aspect of newer technology working with autonomous vehicles is collision avoidance systems. These systems have been implemented on newer UAVs and continue to improve, allowing their the use in tighter confines and spaces. By having a radar-based avoidance signal surrounding the entire UAV, collisions become less likely.
Geofencing is another advancement being implemented into more UAVs to help keep them from intruding into unauthorized spaces, by programming into their computer specific geographic areas that are off limits. UAVs are often also programmed to return to its takeoff location under certain circumstances.
Other technological advances to consider

How much technology do you have in your home and office? Probably more than you realize. While one may immediately think about a smart speaker or home automation system (Alexa, Echo, Nest, etc.), other components offer simple yet productive solutions.
Remote control systems enable you to check whether your doors are locked and your garage door is shut. If not, a touch of a button does the job. Motion sensors enable you to detect intruders around and inside the house, of course. Environmental sensors now monitor for water leaks, moisture and gas/carbon monoxide and provide alerts. How about home automation that utilizes robotic technology? The Roomba vacuum, automatic pool cleaners, and even window washing systems activated when dirt is recognized on your exterior windows are just some of the robotic devices in the modern home.
Precision agriculture utilizes autonomous vehicle control to increase the precision of planting, spraying and harvesting crops. This increase in efficiency has led to higher yields and lower operating costs for the equipment. Another market starting to see more interest is the robotic lawn mowers that functions like the Roomba vacuum. While significantly more expensive than manual mowers, they offer features that can be considered for trade-offs for your time. Depending on your location and needs, they can be set on timers to run day or night and return to base when their battery runs low.
Adapting today’s technology to tomorrow’s surveying tasks
Another relevant technology that does not fit into any of the topics above is the inertial measurement unit (IMU). These sensors are now routinely paired with GNSS receivers in UAVs to help them compensate for pitch and roll. Because of their small form factor, IMUs will increasingly be incorporated into other measurement devices.

It is also safe to say that more handheld devices and smartphones will include lidar scanning capability, as the iPhone 12 Pro and iPad Pro already do. Application and software developers are writing code to make use of data from these devices, so plan on other hardware makers following Apple’s lead.
Voice and motion control will continue to be integrated into data collectors and workstations. By minimizing physical entries into an input system, computers will begin to recognize patterns and automate procedures to increase efficiencies. Programmable voice commands during field data collection will activate various procedures (for instance, specific roadway cross sections or curb island locations) and walk the user through a predetermined set of steps. The possibilities are endless, but we should prepare to take advantage of the technology.
Enticing future generations into a geospatial career

A Geo-spatial career is so much more than just being a surveyor. Our profession needs bright minds who see the world differently. What does that mean?
Most surveying and mapping tasks used to produce 2D deliverables on paper. Today’s geospatial technicians fly UAVs, use point clouds, draft existing conditions in 3D, and analyze data for future applications. By applying what they are learning with new devices, technologies and software platforms, our younger generations can help the surveying and geospatial profession evolve into a data-rich environment that helps facilitate change for our planet. These efforts can help with climate change, provide better data for our communities, and bring societies back together.
Our profession is much more than gathering data; it is helping to make our world a better place through better data analysis and knowledge. Who would not want that?